As you probably have noticed, we have made some considerable progress on the presentation of the documentation on the rsyslog site. Also, the site itself received some upgrades.
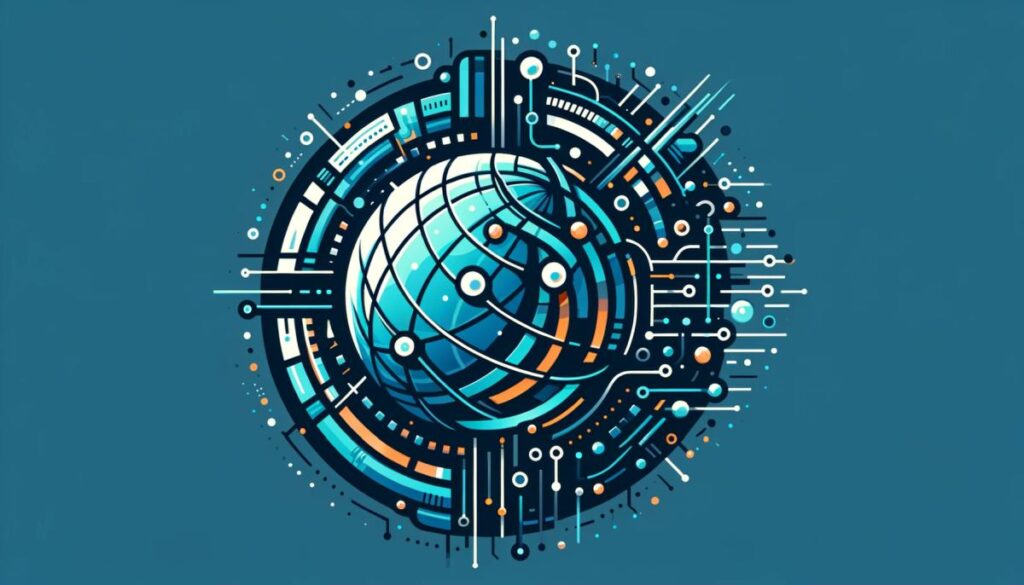
Tech Enthusiast. Nature Lover. Computer Geek. rsyslog lead developer.
As you probably have noticed, we have made some considerable progress on the presentation of the documentation on the rsyslog site. Also, the site itself received some upgrades.
The current state of rsyslog documentation and its representation on our official website has been a subject of concern within the professional community. We are initiating a comprehensive project aimed at systematically addressing these issues. Over the coming weeks, stakeholders can expect a series of methodical changes, some of which may be significantly transformative.
Trying to debug rsyslog.conf issues? This can be hard. Learn here why, and how you can avoid problems. Did you know? Upon startup, rsyslog reads its config file, usually located in /etc/rsyslog.conf. While doing so, it can include config snippets usually located in /etc/rsyslog.d. But no matter if given directly inside the rsyslog.conf, or inside a snippet, the overall config is just a single text document made up of the main config file and the snippets. This is important to note when debugging rsyslog problems.
Yesterday, we held the first open meeting for the (r)syslog community. It was announced on very short notice, but we still had more than 10 participants. Even more important, we had some great discussions. So I call the concept a “success” and plan for more to come.
For everyone who could not attend, here is the meeting recording:
Please accept YouTube cookies to play this video. By accepting you will be accessing content from YouTube, a service provided by an external third party.
If you accept this notice, your choice will be saved and the page will refresh.
I am thinking quite a while about doing online seminars or meetings for folks interested in rsyslog. After some prep work, I decided to do an experiment and invite all of you to the rsyslog community’s first open online meeting. It will be held at 2020-09-29 at 3p UTC (5p CEST, 11a EDT, 8a PDT). The meeting URL is https://meet.rainer-gerhards.de/syslogOpenMeeting. Meeting language is English.
This is more than a community experiment. I have worked on setting up a decent self-hosted Jitsi Meet server (some background info in German). The meeting is also meant as one of the first test runs for this system. So it definitely helps if you are a bit adventurous when you attend.
Continue reading “Experiment: an open online meeting for rsyslog folks”A lot of things are going on, but I also wanted to share news that we have just released a new version of the rsyslog Windows agent. It’s a minor bug-fixing release, so usually no need to rush.
Do you wonder if traveling and vacation season has some effect on Corona spread? German numbers suggest it has – and it can be seen in new cases.
While we have released rsyslog 8.2008 today, binary packages are not yet updated. They will follow follow “a bit” later.
The rsyslog project usually publishes binary packages for many distributions alongside the regular (source) scheduled stable releases. So far, this was a mostly manual process. In the past couple of week, we have worked on a CI system for package build as well as additional automation. We have not yet fully reached our goals, but things look pretty well.
Continue reading “rsyslog 8.2008 released – packages delayed”Librelp 1.7 brings some memory leaks fixes, support for chained certificate files and a bit more. The official release is tomorrow. It will be co-released with rsyslog 8.2008.
The memory leak is especially relevant for some load-balancers, including AWS. I highly recommend to use the new library in such environments. As a side-note, we have also finally done some library symbol cleanups. If you used non-API functions that will hurt you. However, to the best of my knowledge nobody does such dirty tricks.
Adiscon will also integrate the new librelp in to it’s Windows products (including WinSyslog and EventReporter)
Librelp is library for reliable network event logging, It provides syslog-like functionality with a drastically reduced risk of messages loss. Note that industry-standard TCP syslog is unreliable by design. Librelp was written to address these shortcomings.
Rainer Gerhards initiated the librelp open source project. It is primarily sponsored by Adiscon.
Did you search for “rsyslog template variables”? And landed here? Many folks do, so let me explain where you actually find them. TLDR: find them in the property doc.